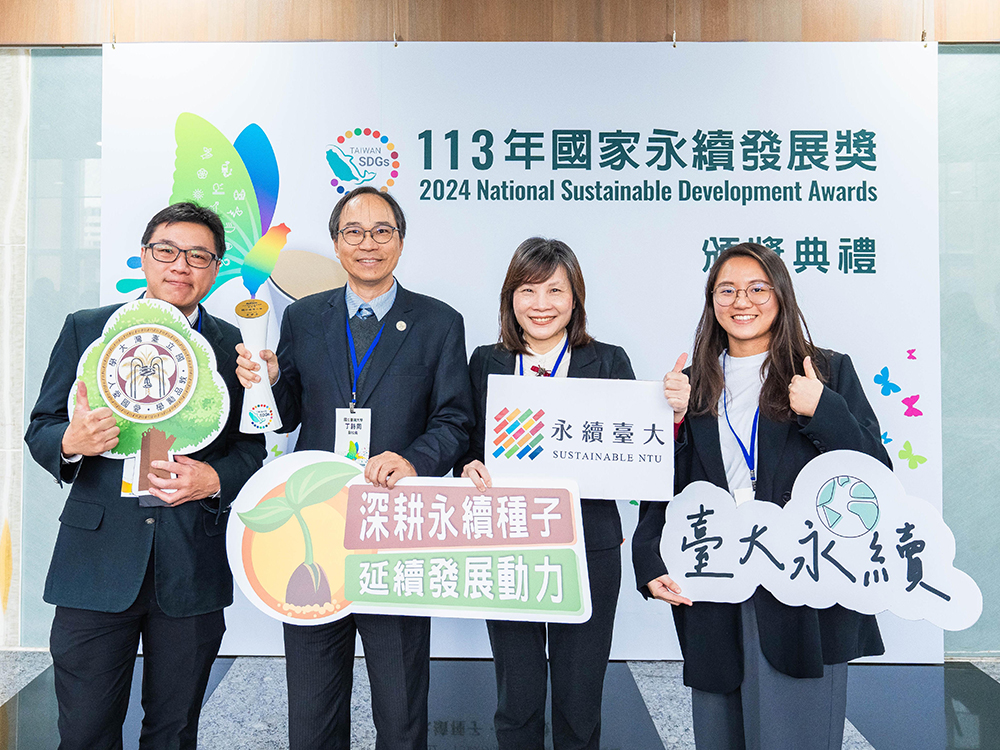
NTU wins the 2024 “National Sustainable Development Awards” for exerting influence in higher education
瀏覽器版本過舊,或未開啟 javascript
請更新瀏覽器或啟用 javascript
Spotlights
Prof. Juang (first from right) and his students. Despite extensive work, our understanding of the underlying principles that guide the “design” of eggs as a load-bearing structure remains incomplete, especially over the broad taxonomic scales. Prof. Juang teamed up with Taipei Zoo, and analyzed eggs of 463 bird species in 36 orders across five orders of magnitude in body mass. The results were published in the journal Scientific Reports.
Astonishing variety of sizes and shapes of avian eggs. Comparison of 14 representative eggs. What do they have in common? Scale bar: 100 mm. Left to right: Blue-tailed bee-eater (Merops philippinus), Javan myna (Acridotheres javanicus), common quail (Coturnix coturnix), rock dove (Columba livia), golden pheasant (Chrysolophus pictus), Blue-and-yellow Macaw (Ara ararauna), Swinhoe's pheasant (Lophura swinhoii), wild turkey (Meleagris gallopavo), Indian peafowl (Pavo cristatus), African penguin (Spheniscus demersus), domestic goose (Anser sp.), red-crowned crane (Grus japonensis), emu (Dromaius novaehollandiae), and ostrich (Struthio camelus). These egg samples were unfertilized or belonged to species with no reproduction need in accordance with the zoo management policy.
Evolution of body mass and C number. A phylogeny of 416 species, studied using computer simulations, for which DNA sequencing data exist. The C number for each species is represented by black line length (linear scale) at branch tips. The red circle indicates the average value (C = 15,000). The branch color indicates the body mass (log scale). Bird images (not to scale) for representative species in each order are shown.
Birds comprise over 10,000 living species, and exhibit an extraordinary diversity in morphology, behavior, and lifestyle traits such as diet, developmental mode, breeding system, and nest type. Modern birds coexisted with nonavian dinosaurs (hereafter “dinosaurs”) during the late Cretaceous period. The dinosaurs were wiped out by the K-Pg mass extinction 66 million years ago, whereas birds not only survived but spread and flourish in every terrestrial habitat on earth. In contrast to the slow incubation (up to 5.8 months) of dinosaurs, such as ornithischian taxa, birds lay relatively small numbers of large eggs with very short incubation periods, ranging from approximately 11 days (e.g., common cuckoos) to 77 days (e.g., wandering albatrosses). Short incubation periods, partially enabled by “contact incubation,” increase survivability by limiting the egg’s exposure to predation and environmental disturbances. However, during contact incubation, the eggshell must be stiff and strong enough to withstand the weight of the incubating bird and the possible impact between eggs, yet weak enough for the chick to break out at hatching. There exist design trade-offs that must be balanced.
This begs a fascinating question: Is there a general “guideline” that dictates the mechanical design of the avian egg for a given body mass? Or what do those diverse avian eggs of over 10,000 bird species have in common as a load-bearing structure? To answer this question, Prof. Jia-Yang Juang (莊嘉揚) of Mechanical Engineering at NTU teamed up with Taipei Zoo. They studied eggs of 463 bird species in 36 orders across five orders of magnitude in body mass, and published the results in the journal Scientific Reports [1].
Stiffness is a key property of load-bearing structures. A fundamental challenge of comparing egg stiffness of different species is that while egg size and shape determine egg stiffness, both vary dramatically across species. (If we regard an egg as a spring, its stiffness is equivalent to the spring constant.) A tiny egg of the 3-g vervain hummingbird (Mellisuga minima) weighed less than 0.3 g, whereas a giant egg of the extinct 450-kg elephant bird (Aepyornis maximus) weighed over 9,000 g. Also, avian eggs come in a variety of shapes: spherical in owls, elliptical in hummingbirds, conical in shorebirds, and other forms with different degrees of asymmetry and ellipticity [2]. Given that the stiffness of an elephant bird egg is much larger than that of a hummingbird egg, whether the egg is large enough to withstand the massive incubating bird remained a question. To answer this question, the team proposed a dimensionless C number to quantify egg stiffness. The C number is characterized by three features. First, it is a simple function of egg stiffness, weight, length, and breadth. Without unit, this dimensionless number enables the comparison of diverse eggs. Second, the parameters required to calculate the C number are easy to measure. For example, stiffness can be measured through standard mechanical compression tests. Weight can be measured using a regular digital scale, and length and breadth a vernier caliper. Third, the C number offers a single metric with clear physical meaning. It indicates the relative stiffness of an eggshell after the effect of egg size and shape is excluded. Moreover, by applying classical shell theory, the C number was found to be directly proportional to the shell’s Young’s modulus and the square of shell thickness, yet inversely proportional to egg weight. In other words, if a particular egg has a relatively large C number, its stiffness is relatively large with respect to its weight, which may be caused by a relatively thicker shell and/or a relatively stiffer material (i.e., larger Young’s modulus).
Prof. Juang and his students conducted experiments on over 500 freshly laid eggs belonging to 40 species from 15 families and 11 orders of birds. (Those egg samples were unfertilized or belonged to species with no reproduction need in accordance with the zoo management policy.) They also developed a computational method, by which they calculated the C numbers of 430 species, which accounted for 36 of all the 40 orders of birds. They created egg models using published egg images and data, and simulated compression tests on computer without the need to acquire freshly laid eggs. After analyzing over 1,000 eggs, the team found that the C numbers of most species fell within a small range centered at 15,000, and the experiments and computer models gave consistent results. Hence, C = 15,000 may be regarded as a “design rule” of avian eggs. In other words, an egg’s weight, shell thickness, and Young’s modulus must satisfy this relationship for the egg to be considered “normal.” An overly large or small C number suggests that the corresponding species may have special reproductive strategies. For example, megapodes (Macrocephalon maleo), kiwis (Apteryx australis), and wandering albatrosses (Diomedea exulans) have C numbers that are substantially smaller than the norm, and all the three have unique reproductive strategies. Kiwis lay enormous eggs (about 20% of the body mass, compared with 5%, a more typical value). Megapodes are the only birds that do not practice contact incubation, relying instead on environmental heat to incubate their eggs. Wandering albatrosses have the longest incubation period of all birds (approximately 77 days, compared with 40 days for ostriches and 21 days for chicken).
Furthermore, Prof. Juang and his students mapped the body masses and C numbers of 416 species (35 orders) onto a recent comprehensive phylogenetic tree. They found that although the evolutionary pattern of body mass is complicated, the C number remains largely constant, suggesting that the “mechanical design” of avian eggs has been well preserved throughout the evolutionary process.
Similar allometric invariances are ubiquitous in nature. Examples include the urination time of large animals [3], the Young’s modulus to density ratio in green woods [4], and the height of a jump [5]. The existence of such invariances is mainly driven by the predominant force on earth--gravity. Given the need to redesign the entire system whenever body mass changes, either through ontogeny or speciation, certain deviations from the general trend, and even exceptions, will occur. However, as body mass varies across many orders of magnitude, these design guidelines are followed with remarkable precision.
Prof. Juang and Pinyi (Penny) Chen (陳品衣), then a junior undergraduate student, conceived the study in September 2014. Starting from scratch, they conducted preliminary experiments using chicken eggs, and worked out the definition of the C number. Chen received a research grant for undergraduates from the Ministry of Science and Technology of Taiwan (MOST 104-2815-C-002-141), and later won the Second Place of the Student Best Paper Award at the 39th National Conference on Theoretical and Applied Mechanics in 2015. Chen graduated from NTU in 2016, and is pursuing her master’s degree in Massachusetts Institute of Technology (MIT). Prof. Juang’s three master’s students, Da-Chang Yang (楊達璋), Shang-Ping Wu (吳尚平), and An Yen (顏安), have continued the study since then. Yang and Yen finished their theses in 2017, and are working as a research engineer and an international exchange student at the Precision Machinery Research & Development Center and Nagoya University, respectively. Yang performed most of the experiments and numerical simulations, and contributed equally to this work with Pinyi. Another coauthor of the paper is Assistant Researcher Hsin-I Hsieh (謝欣怡) of Taipei Zoo.
Link of the paper: https://www.nature.com/articles/s41598-017-14552-0
PDF version: http://rdcu.be/xK6F
DOI: 10.1038/s41598-017-14552-0
References
[1] J.-Y. Juang, P.-Y. Chen, D.-C. Yang, S.-P. Wu, and H.-I. Hsieh, “The avian egg exhibits general allometric invariances in mechanical design,” Sci. Rep., pp. 1–11, 2017.
[2] M. C. Stoddard, E. H. Yong, D. Akkaynak, C. Sheard, J. A. Tobias, and L. Mahadevan, “Avian egg shape: Form, function, and evolution,” Science, vol. 356, no. 6344, pp. 1249–1254, 2017.
[3] P. J. Yang, J. Pham, J. Choo, and D. L. Hu, “Duration of urination does not change with body size.,” Proc. Natl. Acad. Sci. U. S. A., vol. 111, no. 33, pp. 11932–11937, Jun. 2014.
[4] T. Mcmahon, “Size and shape in biology,” Science, vol. 179, no. 1973, pp. 1201–1204, 1973.
[5] T. A. McMahon and J. T. Bonner, On Size and Life, 1st editio. Scientific American Books - W. H. Freeman & Co., 1983.
NTU wins the 2024 “National Sustainable Development Awards” for exerting influence in higher education
Prof. Chih-Kung Lee of NTU’s Institute of Applied Mechanics elected NAI Fellow
NTU Launches Startup Initiatives at Zhubei Campus
2024 NTU-KU-UHH Trilateral Symposium: Uniting for Sustainable Future and Next Generation
Signing Ceremony for the donation of “Next-Generation Smart 3D Geospatial Information Center” at NTU Zhubei Campus
Current Spotlights